Speakers
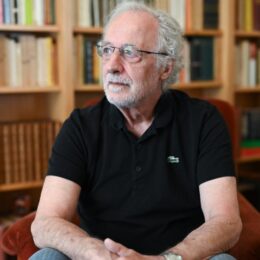
Ionization of argon by an attosecond pulse train (APT) yields electron wavepackets (WP) which can be accelerated in the field of a NIR laser, return to the parent ion and extract a second electron by an e2e collision. The ionization time is controlled with attosecond precision and the doubly charged ion signal monitored as a function of the delay between the APT and the laser optical cycle. Considering the wavepacket spreading and the e2e dependence on the electron kinetic energy, allows to predict the time dependence of the doubly charged ion seen in the experiment. This is, I believe, the first application of an APT in the time domain. In addition, adjusting the release time of the WP allows to control the trajectory, while spectrally sculpting the APT allows to mimic a tunneling ionization, and improve our understanding of the strong field recollision physics.
Authors: Andrew J. Piper, Qiaoyi Liu, Abraham Camacho Garibay, Dietrich Kiesewetter, Vyacheslav Leshchenko, Jens E. Bækhøj, Pierre Agostini, Kenneth J. Schafer, Louis F. DiMauro, Yaguo Tang.
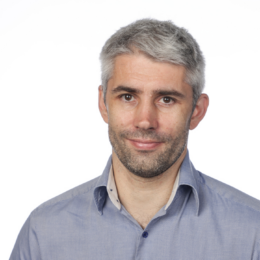
Cavity quantum electrodynamics offers unique perspectives for quantum simulations. It allows for real-time, continuous, weakly destructive monitoring of the dynamics of atoms inside the cavity mode, as well as for photon-mediated long-range interactions.
In this talk, I will present the real-time observation of the dynamics of a Fermi gas undergoing a density-wave phase transition induced by cavity-mediated interactions. There, we track the exponential rise of the order over several orders of magnitude and connects its rate to the microscopic physics of the Fermi gas. We find that the growth rate is insensitive to the contact interaction strength from the ideal gas up to the unitary limit and can exceed the Fermi energy by an order of magnitude, in quantitative agreement with theory. Our results generalize to linear interaction ramps, where deviations from the adiabatic behavior are captured by a simple dynamical ansatz.
I will then present a new generation of observation methods that combine cavities with microscope techniques. I will first show that cavity-induced density wave order can be directly observed in-situ through high-resolution absorption imaging. Second, I will introduce and describe a new ‘cavity-microscope’ device that combines in a single device and a single optical axis a microscope and a cavity. This device allows for high resolution spatial control of light-matter interaction, opening the perspective of programing interactions in space and time to realize a quantum simulations of the Sachdev-Ye-Kiatev model.
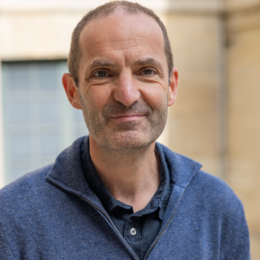
This talk will present our recent work on the control of interactions between cold atoms to implement spin Hamiltonians useful for quantum simulation of many-body problems, or quantum optics situations. We rely on laser-cooled atomic ensembles of Rb, consisting either of individual atoms in tweezer arrays, or dense elongated atomic gases.
By exciting arrays of up to 100 atoms into Rydberg states, we make the atoms interact by the resonant dipole interaction. The system implements the XY spin ½ model. When the system is placed out of equilibrium, the interactions generate scalable spin squeezing [Bornet et al., Nature 2023]. Analyzing the spread of correlations across the system, we measure the dispersion relation and observe the predicted anomalous behavior in the ferromagnetic case, a consequence of the dipolar interactions [Chen et al., arXiv:2311.11726].
Using an elongated dense atomic ensemble driven on an optical transition, we rely on the collective coupling of many atoms to a single mode of the electromagnetic field to observe driven superradiance and demonstrate the generation of non-gaussian light [Ferioli et al., PRL 2024].
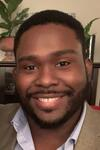
Quasicrystals are aperiodic yet exhibit long-range order, without translation symmetry but with rotational symmetries that are mathematically forbidden in periodic lattices. In 1984, Shechtman performed X-ray diffraction measurements on a metallic alloy, revealing 10-fold rotational symmetry in the diffraction pattern. This work eventually led to the redefinition of what constitutes a crystal, and the recognition of the reality of aperiodic crystals. Shechtman was then awarded the 2011 Nobel prize in chemistry for the discovery of aperiodic crystals.
In recent decades, band structure and its interplay with topology has provided deep insight into intriguing behavior in periodic crystalline quantum materials. However, thirty years after the discovery of aperiodic crystals, the role of the energy spectrum and its interplay with topology is not well-understood for quasicrystals because standard theoretical methods used to study the energy spectrum of a crystal rely on translational symmetry. Quantum simulation of a quasicrystal would open a window into quasicrystalline “band structure” and topology that is difficult to access with theoretical and analytical methods alone.
This talk will describe the design of an experiment in which a quantum gas is confined within a 10-fold rotation-symmetric quasiperiodic optical lattice, which serves as a quasicrystal quantum simulator.
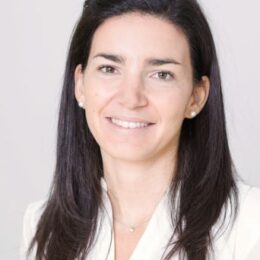
Attosecond science is nowadays a well-established research field, which offers formidable tools for the realtime investigation of electronic processes. In this context, we have demonstrated that attosecond pulses can initiate charge migration in aromatic amino-acids [1] as well as in the DNA nucleobase adenine [2]. These pioneering investigations have been done in ionized molecules and there is still a long path towards attochemistry and the control of the reactivity of neutral molecules via electronic coherences
Here, I will show our most recent work devoted to the investigation of charge migration in neutral molecules and its applications to manipulate the outcome of photochemical and photophysical processes. We exploited our new light source delivering few-femtosecond UV pulses [3] in order to photoexcite below the ionization threshold and trigger electronic dynamics in the chiral molecule methyl-lactate. We used time-resolved photoelectron circular dichroism (TR-PECD) to image charge migration and disclose – for the first time – its impact on the molecular chiral response. We show that charge migration enables an ultrafast chiroptical switching effect where the amplitude and direction of the photoelectron current generated by PECD can be controlled on a sub-10 fs timescale [4]. These results provide important perspectives to exploit charge-directed reactivity for controlling the chiral properties of matter at the electron time scale
[1] F. Calegari et al., Science 346, 336-339 (2014).
[2] E. P. Månsson et al, (Nature) Commun. Chem. 4, 73 (2021)
[3] M. Galli, et al., Optics Letters 44, 1308-1311 (2019).
[4] V. Wanie et al., Nature (2024) DOI: 10.1038/s41586-024-07415-y
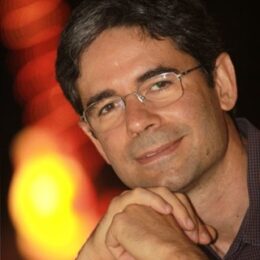
I describe work with trapped antihydrogen at the ALPHA collaboration at CERN. The 1S-2S transition frequency measurement[1] used a 300 mK average energy sample. The resolution, lower than that of trapped H spectroscopy at MIT[2] and still far from the natural linewidth limit, allowed the most precise comparison of antimatter with matter[3]. With laser cooling[4], the ALPHA experiment data from 2023 (under blind analysis) points to a 13 significant figure absolute frequency measurement. A future comparison at 15 figures or more will be discussed in conjunction with techniques[5] to load hydrogen into the same antihydrogen trap and its detection independent of annihilation signal[6]. I also briefly describe results on the gravitational fall of antihydrogen[7,8].
[1] M. Ahmadi et al. Characterization of the 1S–2S transition in antihydrogen. Nature 557,71(2018)
[2] C.L.Cesar et al. Two-photon spectroscopy of trapped atomic hydrogen. Phys.Rev.Lett.77, 255(1996)
[3] C.G.Parthey et al. Improved measurement of the hydrogen 1S–2S transition frequency. Phys.Rev.Lett.107,203001(2011)
[4] C.J. Baker et al. Laser cooling of antihydrogen atoms. Nature 592,35(2021).
[5] L.O. Azevedo et al. Adaptable platform for trapped cold electrons, hydrogen and lithium anions and cations. Commun.Phys.6,112(2023)
[6] C.L. Cesar, A sensitive detection method for high resolution spectroscopy of trapped antihydrogen, hydrogen and other trapped species. J.Phys.B 49,074001(2016)
[7] E.K. Anderson et al., Observation of the effect of gravity on the motion of antimatter, Nature 621, 716(2023)
[8] C.L.Cesar, Trapping and spectroscopy of hydrogen. Hyp.Interact.109,293(1997)
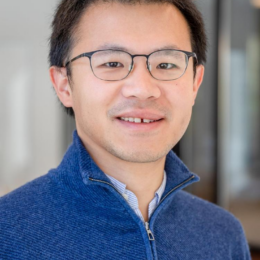
Ultracold polar molecules, with their rich internal structure and tunable long-range interactions, have long been proposed as a platform for quantum science. In particular, programmable arrays of molecules individually trapped in optical tweezers promise to be a powerful new platform for quantum simulation and quantum information processing.
In this talk, I will report several advances from our group on the quantum control of laser-cooled molecules held in programmable optical tweezer traps. In the first part of the talk, I will report our demonstrations of creating defect-free molecular arrays, observing coherent interactions between molecules, deterministically entangling molecules, and controlling their motional states at the quantum level. These establish the basic building blocks for quantum simulation and information processing. In the second part of the talk, I will report on quantum measurement and feedback in molecules. In particular, I will report the first demonstration of classical and quantum erasure error detection in molecules, which are both important capabilities for quantum science with molecules. Our work on classical erasure error detection and their correction allows robust high-fidelity internal state preparation, which directly opens to preparation of large-scale systems needed for quantum simulation in the many-body regime. Our work on quantum erasure errors demonstrates a key capability for quantum error correction, as erasure conversion has recently been found to vastly increase fault-tolerant thresholds in quantum error correcting codes.
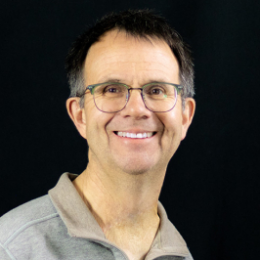
The past three years have seen three dipole-moment measurements of record-breaking accuracy – the magnetic dipole moments (aka “g-2”) of the muon and of the electron, and the electric dipole moment (EDM) of the electron. I will focus in on the latter measurement, performed at JILA. Then I will compare and contrast the relative implications of all these three measurements for the search for Beyond Standard Model physics.
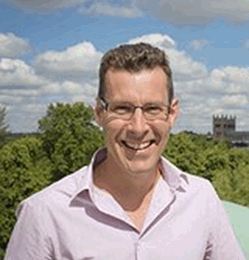
Ultracold polar molecules are an exciting new platform for quantum science and technology. The combination of rich internal structure of vibration and rotation, controllable long-range dipole-dipole interactions and strong coupling to applied electric and microwave fields has inspired many applications. These include quantum simulation of strongly interacting many-body systems, the study of quantum magnetism, quantum metrology and molecular clocks, quantum computation, precision tests of fundamental physics and the exploration of ultracold chemistry. Many of these applications require full quantum control of both the internal and motional degrees of freedom at the level of single molecules, combined with traps that support long coherence times for rotational-state superpositions.
Using ultracold RbCs molecules assembled from ultracold atoms, we demonstrate all these requirements. We present a novel magic-wavelength trap that supports second-scale rotational coherences in a gas of molecules and gives access to controllable dipole-dipole interactions. We also report the efficient assembly of individual molecules in optical tweezers. Using mid-sequence detection of molecule formation errors, we demonstrate rearrangement to produce small defect-free arrays. By transferring the molecules into magic-wavelength tweezers, we demonstrate long-lived rotational coherences that will enable spin-exchange interactions between molecules.
Finally, as an outlook, we demonstrate a new hybrid platform that combines single ultracold molecules with single Rydberg atoms, opening up the prospect of non-destructive readout of the molecular state and fast entangling gates.
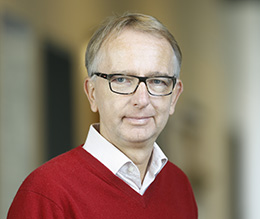
Combining ultrafast physics with matter wave diffraction, we demonstrate how electron wave packets from photoionization in an ultrashort laser pulse are diffracted by a time-delayed ultrashort pulsed standing light wave. This exploits the Kapitza-Dirac effect to create a movie of the phase evolution of a freely moving electron1.
In another example we show the time evolution of the wavefunction of a free moving atom released from an ultracold He molecule2 using multi coincidence detection with a COLTRIMS reaction microscope
1 Lin et al Science 6690, 1467 (2024)
2 Kunitiski et al Nat. Phys., 17, 174 (2021)
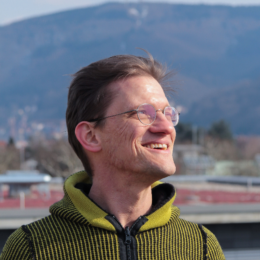
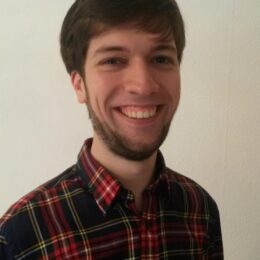
Ultracold polar molecules have long been regarded as a powerful platform for quantum many-body physics and quantum simulation.
Realizing these applications however requires cooling to quantum degeneracy and simultaneously the ability to control interactions.
In this talk I will discuss how microwaves can be used to engineer repulsive interactions between molecules that “shield” them from collisional loss.
Controlling collisions between ultracold molecules has recently enabled their efficient evaporative cooling to Fermi[1] and Bose[2] degeneracy.
In addition, microwave shielding offers full control of the contact and dipole-dipole interactions, realizing a new platform for dipolar quantum matter.
[1] Schindewolf et al. Nature 607, 677–681 (2022)
[2] Bigagli et al. arXiv:2312.10965
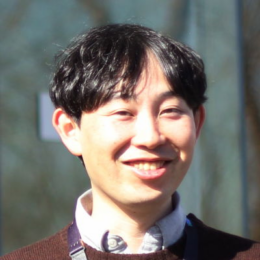
Optical clocks exhibit better performances in terms of frequency stability and accuracy compared with microwave Cs fountain clocks, and are considered as promising candidates for a redefinition of the SI second. However, the robustness of optical clocks has not generally reached to a level of Cs fountain clocks that are running nearly continuously for a long period. At National Metrology Institute of Japan (NMIJ), we have developed an Yb optical lattice clock NMIJ-Yb1 which can be operated for many months with an uptime of > 80 %. Our best operation records include uptimes of 80.3 % for half a year from October 2019 to March 2020, 94.5 % for 30 days in August 2021, and 97.0 % for 20 days in March 2022. We here present some technical details about the robustness of NMIJ-Yb1 and its applications to (i) the frequency calibration of International Atomic Time (TAI) with reduced link uncertainties, (ii) generation of a stable local time scale by steering a hydrogen maser with NMIJ-Yb1, and (iii) search for ultralight dark matter candidates by high uptime comparisons between NMIJ-Yb1 and our Cs fountain clock NMIJ-F2.
This work was supported by Japan Society for the Promotion of Science (JSPS) KAKENHI Grant Number JP17H01151, JP17K14367, JP18K04989, JP22H01241, JST-Mirai Program Grant Number JPMJMI18A1, and JST Moonshot R&D Program Grant Number JPMJMS2268, Japan.
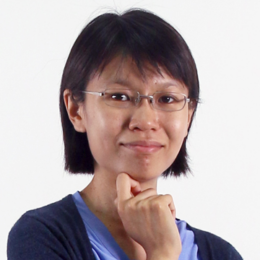
Scalable and programmable Rydberg atom arrays offer a promising platform for exploring quantum science. When atoms undergo Rydberg blockade, the prevention of nearby atoms from being excited to the Rydberg state leads to constrained dynamics, such as that in the effective PXP model. Complementary to the Rydberg-blockade mechanism is facilitation, where a Rydberg atom must be present in order to drive other nearby atoms to the Rydberg state.
In this talk, I will present our results on combining both blockade and facilitation by invoking beyond-nearest-neighbor interactions. Our tools allow us to realize a broad class of models where the Hilbert space has been shattered into exponentially many disjointed subspaces. For strongly fragmented models, we uncover interesting dynamics such as Z2n quantum many-body scarring, which generalizes beyond the Z2 scars previously reported in cold-atom systems. When bringing multiple long-range interactions into resonance, we observe quantum thermalization restricted to Hilbert space fragments. Notably, thermalization between states belonging to different subspaces is forbidden, even when these states have the same energy, defying expectations from the eigenstate thermalization hypothesis.
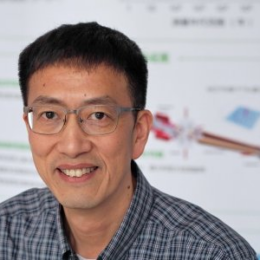
The long-lived noble-gas isotope 81Kr is the ideal tracer for old water and ice with ages of 0.1 – 1 million years, a range beyond the reach of 14C. 81Kr-dating, a concept pursued over the past six decades, is now available to the earth science community at large. This is made possible by the development of the Atom Trap Trace Analysis (ATTA) method, in which individual atoms of the desired isotope are captured and detected. ATTA possesses superior selectivity, and is thus far used to analyze the environmental radioactive isotopes 85Kr, 39Ar, 41Ca, and 81Kr, These isotopes have extremely low isotopic abundances in the range of 10-17 to 10-11, and cover a wide range of ages and applications. In collaboration with earth scientists, we are dating groundwater and mapping its flow in major aquifers around the world, and dating old ice from the deep ice cores of Antarctica, Greenland, and the Tibetan Plateau. For an update on this worldwide effort, please google “ATTA Primer”.
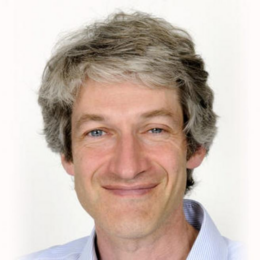
Trapped-ion qubits are one of the leading platforms for quantum computing. Combined with photonic interconnects, trapped-ion processors can form the basis of a quantum network. Our apparatus in Oxford consists of two independent ion traps, separated by about 2 metres, linked via a single-photon optical fibre interface [1]. It is capable of generating entangled pairs of ions, one in each trap, with high fidelity (94%) and at high rates (182/sec). We have previously used it to implement fully device-independent quantum key distribution [2] and entanglement-enhanced comparison of optical atomic clock transitions [3].
Recently we have added robust memory qubits to the nodes [4], enabling the quantum information to be preserved for around 10 seconds, much longer than the time taken to generate remote entanglement. I will present recent work on demonstrating “blind” quantum computing using this hybrid matter-photon system [5], and preliminary results on implementing distributed logic operations across the network link.
[1] L. J. Stephenson, D. P. Nadlinger et al., Phys.Rev.Lett. 124, 110501 (2020).
[2] D. P. Nadlinger, P. Drmota et al., Nature 607, 682 (2022).
[3] B. C. Nichol, R. Srinivas et al., Nature 609, 689 (2022).
[4] P. Drmota, D. Main et al., Phys.Rev.Lett. 130, 090803 (2023).
[5] P. Drmota, D. P. Nadlinger et al., Phys.Rev.Lett. 132, 150604 (2024).
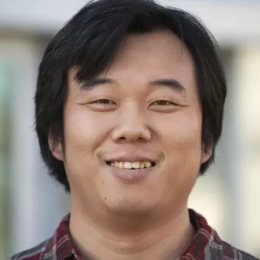
Microwave-shielding has proven to be a powerful technique for producing degenerate quantum gases of polar molecules as well as assembling ultracold polyatomic molecules. Here, I will review our efforts in controlling the interactions of ultracold molecules using microwave fields, enabling us to stabilize the molecular gases and evaporate them to temperatures well below the Fermi temperature. The shape, symmetry, and depth of the intermolecular potential can be flexibly controlled by the polarization, strength, and frequency of the microwave field. This is a unique feature of microwave-shielded polar molecules that is distinguished from ultracold atoms. It allows us to observe field-linked resonances in collisions of polar molecules, providing a universal tool for independently controlling the dipolar and contact interactions of molecules, as well as creating exotic long-range tetratomic molecules. In the end, I will show our progress towards a p-wave superfluid of dimers and its crossover to a Bose-Einstein condensate of tetramers.
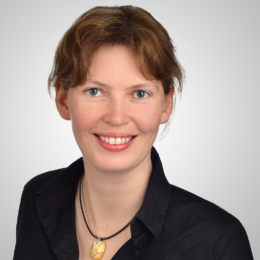
Trapped and laser-cooled ions allow for a high degree of control of atomic quantum systems. They are the basis for modern atomic clocks, quantum computers and quantum simulators. In our research we use ion Coulomb crystals, i.e. many-body systems with complex dynamics, for precision spectroscopy. Multi-ion clocks will not only improve the stability by exploiting the higher signal to noise of multiple ions or their uncertainty by allowing for sympathetic cooling of clock ions using a separate ion species but will be the basis for future entangled clocks and cascaded clocks.
This paves the way to novel optical frequency standards with ultra-high stability reaching 10-19 relative accuracy and stability, and for applications such as relativistic geodesy and quantum simulators in which complex dynamics becomes accessible with atomic resolution. We will report on the first multi-ion clock operation and frequency comparisons.
Last but not least, I will briefly discuss new world-record limits we obtained in our work on an improved test of local Lorentz invariance using 172Yb+ ions and the search for new bosons using clock spectroscopy on even Yb+ isotopes.
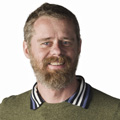
Dipolar quantum gases are offering an unprecedented opportunity to explore the supersolid, a quantum phase intermediate between ordinary superfluids and crystals that might be present in many superfluids and superconductors. The main property characterizing supersolids, a sub-unity superfluid fraction, is still elusive fifty years after it was proposed by A. J. Leggett.
I will show how the superfluid fraction of a dipolar supersolid can be measured directly by exciting Josephson oscillations in the supersolid lattice, and measuring the corresponding tunnelling energy. The measured superfluid fraction spans a relative large range between one and zero by varying the interaction strengths, and is in agreement with the original theory predictions. The very existence of spontaneous Josephson oscillations confirms supersolids as a separate quantum phase of matter.
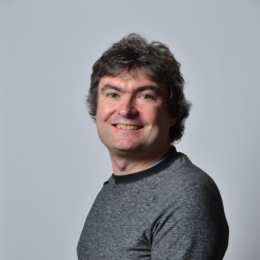
Atom interferometers are versatile instruments, working as quantum sensors in the field and probing the laws of physics at the deepest level in the lab. Examples are testing the standard model by measuring the fine-structure constant and searching for quantum aspects of the gravitational field, such as superposition and entanglement.
In typical atom interferometers, the freely-falling motion of the atoms limits the free evolution to a few seconds. We will show that atoms trapped in optical lattices allow free evolution times as long as 70 seconds, during which small effects can generate large, measurable phase shifts. We use a system of signal inversions to suppress systematics caused by the trapping potential. This enables measuring the attractive force from a small tungsten mass with a precision that is five times improved over a previous measurement with freely falling atoms. The long-lasting coherence lends itself well to measuring the dynamic interactions between the atoms and a coherent mechanical system, such as a torsion pendulum. This may yield insights into the coherence of the gravitational field itself. A setup that is intended to take the first step is under construction.
Our measurement of the fine-structure constant uses atoms in free fall. This isolates the atoms strongly from environmental influences and minimizes systematic effects. It is favorable in applications that require long-term stability or absolute accuracy. Minimizing the leading systematic effects requires clean and well characterized laser wavefronts. This is hoped to improve the accuracy beyond current limitations and clarify the issues raised by the current, discrepant, measurements.
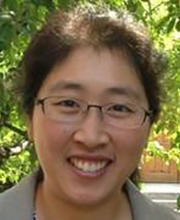
Coherence and entanglement are key features of quantum mechanics, although they are susceptible to environmental perturbations. A conventional strategy to entangle qubits with high fidelity is to leverage precisely controlled interactions while keeping qubits from decohering. By leveraging electric dipolar interactions, I will report entangling individual trapped NaCs molecules in optical tweezers. Going beyond this paradigm, we explore and ask the question: Can coherence be preserved during chemical reactions and subsequently harnessed to produce entangled products? To address this question, we conduct investigations within the context of an atom-exchange chemical reaction (2KRb -> K2 + Rb2) at a temperature of 500nK. I will share our research findings including surprises and puzzles.
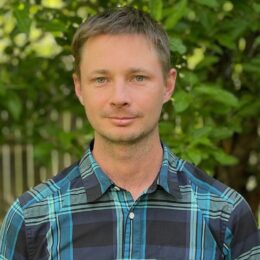
A quantum computer capable of useful computation will likely require numbers of qubits beyond what is accessible today, a means of performing mid-circuit readout of a subset of those qubits, and the ability to perform high-fidelity gates. In this talk, I will present recent progress towards achieving these goals at Atom Computing Inc, using neutral ytterbium atoms confined within arrays of optical tweezers. This includes an iterative approach to assembling and maintaining arrays of atoms, a means of selective qubit readout through local light-shifts, and a demonstration of high-fidelity two-qubit gates using a protocol suited to the level structure of alkaline earth atoms.
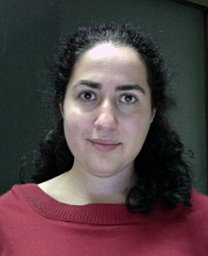
Monitoring electron dynamics in matter requires the use of coherent light sources that can offer attosecond resolution. For more than two decades, attosecond experiments have been mostly performed using table-top experimental set ups where trains of attosecond pulses are produced through high-harmonic generation (HHG). But the most recent technological developments at free electron lasers (introducing, for instance, self-amplified spontaneous emission schemes) also allows one nowadays to produce phase-controlled pulses that offer the necessary time resolution to access and steer electronic motion in matter. This talk presents our latest progresses on state-of-the-art ab initio theoretical methods that are employed to describe ongoing experiments and forthcoming applications of attosecond pump-probe spectroscopic techniques to investigate the ultrafast electron dynamics in gas phase atomic and molecular targets. In particular, we investigate, using nearly-exact calculations, the role of the electron-electron and electron-nuclear correlation terms in laser-induced excitation and single and multiple ionization events.
Website
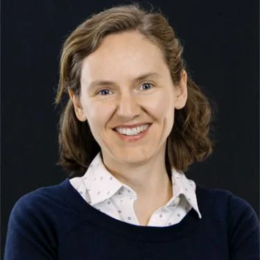
Scalable Rydberg atom arrays are a fast evolving platform for programmable quantum computation and simulation. We present a new system for the control of 2D Rydberg atom arrays embedded in a cryogenic environment. Our high optical access system is compatible with long vacuum lifetime, high-fidelity atomic manipulation, and reduction of blackbody-driven Rydberg decay. I present measurements of ground-state and initial Rydberg manipulation in our cold box, as well as long-lived atoms in a cryopumped vacuum with which we study single-atom imaging of rubidium with high survival, an important component of high-fidelity atom rearrangement. I discuss plans to harness similar long-lived vacuum conditions for addressing one source of loss and heating in Fermi gases. I also outline our ongoing efforts in controlling external degrees of freedom in an optical tweezer traps in the context of cooling, light-assisted collisions, and non-classical motional states.
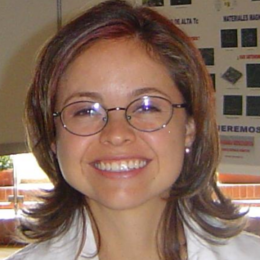
Dipolar interactions provide opportunities for the exploration of many-body phenomena that remain difficult to be seen in systems with just contact interactions. One such phenomenon falls under the general heading of itinerant quantum magnetism, where magnetic moments (spins) interact with one another with coupling strength J as they hop in a periodic potential at a rate t. Their dynamics is described by the so-called t-J model, a model originally emerging from the large interaction energy expansion of the Hubbard Model, which is believed to describe the fundamental physics behind high temperature superconductors. In this talk I plan to report on the first realization of a generalized t-J spin model with dipolar interactions using a system of ultracold KRb fermionic molecules with a spin-1/2 encoded in the two lowest rotational states. Our study paves the way for future explorations of itinerant spin dynamics and quantum magnetism with highly tunable molecular platforms in regimes challenging for existing numerical and analytical methods that could shed light on the complex behaviors in real materials.
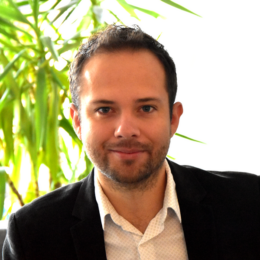
Rapid progress in the experimental control and interrogation of molecules is enabling new opportunities for investigating the fundamental laws of our universe. In particular, molecules containing heavy, octupole-deformed nuclei, such as radium, can offer enhanced sensitivity for measuring yet-to-be-discovered parity and time-reversal violating nuclear properties. This talk will present recent highlights and perspectives from laser spectroscopy experiments on these species, as well as discuss the relevance of these experiments in addressing open problems in nuclear and particle physics.
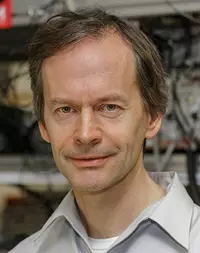
Trapped ions illuminated with laser light constitute an engineered quantum system that enables the realization of spin-lattice models with long-range interactions. While linear ion strings of up to 50 ions have been used for this purpose for a long time, experiments with two-dimensional ion crystals held in radiofrequency traps started only recently.
I will present experiments demonstrating control over planar ion crystals with more than 100 ions [1]. After cooling all transverse motional modes of the crystal close to the ground state, long-range transverse field Ising models can be realized by Raman interactions that off-resonantly couple the Zeeman ground states of singly-charged calcium ions to crystal’s motional modes. We demonstrate the creation of spin-squeezed states of up to 91 ions using an approach previously realized with long ion chains [2], characterize the spin-spin interactions and demonstrate single-qubit control over all ions in the crystal.
[1] D. Kiesenhofer, H. Hainzer et al, PRX Quantum 4, 020317 (2023)
[2] J. Franke et al., Nature 621, 740 (2023)
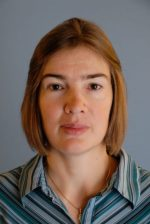
The extraordinary advances in quantum control of matter and light have been transformative for atomic and molecular precision measurements enabling probes of the most basic laws of Nature to gain a fundamental understanding of the physical Universe. Exceptional versatility, inventiveness, and rapid development of precision experiments supported by continuous technological advances and improved atomic and molecular theory led to rapid development of many avenues to explore new physics. The development of high-precision optical atomic clocks enables searches for the variation of fundamental constants, dark matter, violations of Lorentz invariance, and tests of gravity. Deployment of high-precision clocks in space will open the door to new applications, including precision tests of gravity and relativity, searches for a dark-matter halo bound to the Sun, and gravitational wave detection in wavelength ranges inaccessible on Earth, and others.
I will give a broad overview of atomic clock applications on Earth and in space, focusing on searches for physics beyond the standard model of elementary particles. Several examples will be highlighted, including dark matter searches with atomic and nuclear clocks and new ideas for searches of physics beyond the standard model with quantum sensors in space. New ideas for detection of transient signals will be presented.
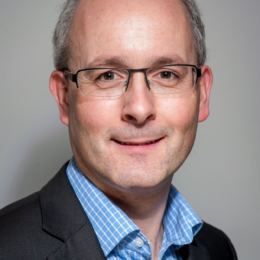
The extreme electronic properties of highly charged ions (HCI) render them highly sensitive probes for testing fundamental physical theories. The same properties reduce systematic frequency shifts, making HCI excellent optical clock candidates [1]. The technical challenges that hindered the development of such clocks have now been overcome, starting with their extraction from a hot plasma and sympathetic cooling in a Paul trap [2], readout of their internal state via quantum logic spectroscopy [3], and finally the preparation of the HCI in the motional ground state of the trap [4]. Here, we present the first optical clock based on an HCI (Ar13+ in our case) and a full evaluation of systematic frequency shifts [5]. The achieved uncertainty is almost eight orders of magnitude lower than any previous frequency measurements using HCI and comparable to other optical clocks. By measuring the isotope shift between 36Ar13+ and 40Ar13+ the theoretically predicted QED nuclear recoil effect could be confirmed. Finally, first results on the search for a 5th force based on isotope shift spectroscopy of Ca+/Ca14+ isotopes will be presented. This demonstrates the suitability of HCI as references for high-accuracy optical clocks and to probe for physics beyond the standard model.
References
- Kozlov, M. G. et al., Rev. Mod. Phys. 90, 045005 (2018).
- Schmöger, L. et al., Science 347, 1233 (2015).
- Micke, P. et al., Nature 578, 60 (2020).
- King, S. A. et al., Phys. Rev. X 11, 041049 (2021).
- King, S. A. et al., Nature 611, 43 (2022).
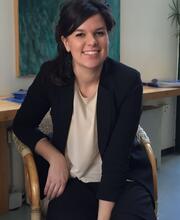
In this talk, we will explore recent advancements in quantum science using Rydberg atom arrays and present future applications enabled by the use of a dual-species array based on a mixture of alkali and alkaline-earth atoms. Trapped arrays of interacting Rydberg atoms have become a leading platform for quantum information processing and quantum simulation due to their large system size and programmability. The use of two atomic species allows for the independent control of two different sets of qubits for quantum error correction, and selective tuning of inter- and intra-species interactions for more flexible Hamiltonian engineering. These new features enable more efficient protocols for quantum information processing and would allow to simulate a broader class of highly-entangled phases of matter. We will present the current development of a new experimental platform based on Yb and Rb atom arrays, leveraging the distinct characteristics of these two atomic species to create a versatile platform for quantum simulation and quantum information processing.
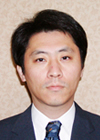
We report our recent study of the precision measurement towards new physics beyond the Standard Model. We perform precision isotope-shift measurements for ultra-narrow optical clock transitions of four bosonic isotope pairs of ytterbium atoms loaded in a three-dimensional magic wavelength lattice. For the 1S0 and 3P0 clock transition, we achieve the part-per-billion precision [1]. In addition, we observe a new clock transition between the 1S0 and 4f135d6s2(J=2) states [2], and determine the isotope shifts with less than 10 Hz precision. These results, combined with other precision data using ytterbium atoms and ions, show the significantly large non-linearity of the King relation, and will allow us to obtain a bound of the coupling strength of a new hypothetical particle mediating a force between electrons and neutrons with a generalized King plot approach [3].
[1] K. Ono, et al., Phys. Rev. X 12, 021033 (2022).
[2] T. Ishiyama et al., Phys. Rev. Lett. 130, 153402 (2023).
[3] K. Mikami, et al., Eur. Phys. J. C 77, 896 (2017).
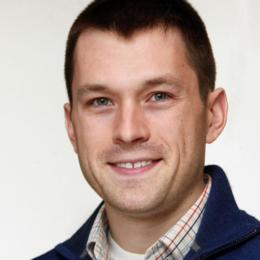
Neutral atom quantum computing is a rapidly developing field. Exploring new atomic species, such as alkaline earth atoms, provides additional opportunities for cooling and trapping, measurement, qubit manipulation, high-fidelity gates and quantum error correction. In this talk, I will present recent results from our group on implementing high-fidelity gates on nuclear spins encoded in metastable 171Yb atoms, including mid-circuit detection of gate errors that give rise to leakage out of the qubit space, using erasure conversion. I will conclude by discussing several new directions including spectroscopy and modeling of 171Yb Rydberg states and interactions, which has led to improved two-qubit gate fidelities.
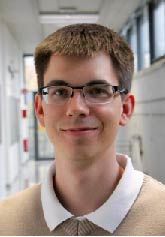
The thorium-229 nucleus has a unique, low-lying isometric state allowing for laser spectroscopic investigations that are otherwise only accessible in electronic transitions. Here, we report on the first resonant laser excitation of the Th-229 nucleus with a tabletop tunable laser system. The laser system consists of two seeded dye amplifiers that are frequency-converted via four-wave mixing in Xenon to the vacuum-ultraviolet (VUV) region. Using this light source with Th-229 doped calciumfluoride crystals we were able to determine the center frequency of 2020.409(7) THz corresponding to 148.3821(5) nm of the nuclear transition. The fluorescence lifetime in the crystal is 630(15) s, corresponding to an isomer half-life of 1740(50) s for a nucleus isolated in vacuum. These results pave the way towards high-resolution nuclear laser spectroscopy of Th-229 and an optical nuclear clock with high sensitivity in fundamental tests.
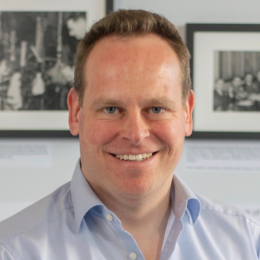
We have recently created the first Bose-Einstein condensate (BEC) of dipolar molecules [1-3]. We efficiently cool sodium-cesium molecules from 700 nK to less than 10 nK, deep into the quantum degenerate regime. The lifetime of the molecular BEC is longer than one second, reaching a level of stability similar to ultracold atomic gases. A cornerstone of this advance is double microwave shielding, a novel technique that gives us control over intermolecular interactions and reduces inelastic loss of molecules by four orders of magnitude. The creation of a BEC constitutes the first observation of a phase transition in an ultracold molecular gas.
In this talk, I will discuss our experimental approach, share latest insights, and give an outlook on opportunities with our system for many-body quantum physics, quantum simulation, and quantum information. Thanks to a large dipole moment, BECs of sodium-cesium molecules promise access to regimes of dipolar quantum matter that have been inaccessible so far.
References:
- Bigagli, Yuan, Zhang, et al., Observation of Bose-Einstein condensation of dipolar molecules, arXiv:2312.10965 (2023) and Nature (2024)
- Bigagli, et al., Collisionally stable gas of bosonic dipolar ground state molecules, Nature Physics, 19, 1579-1584 (2023)
- Stevenson, et al., Ultracold gas of dipolar NaCs ground state molecules, Phys. Rev. Lett. 130, 113003 (2023
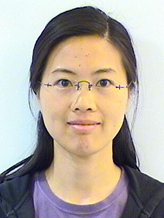
Continuous quantum measurement is an intriguing and challenging problem both theoretically and experimentally because it involves measurement backaction and quantum trajectories. Quantum enhanced sensing in the continuous regime is even more demanding with a requirement of sustained entanglement in the presence of unknown signal perturbations. In this talk, I will show how we perform continuous quantum nondemolition measurements for a hot atomic ensemble of more than 10^10 atoms, and achieve steady state spin squeezing lasting in the lab for over a day. Then, we demonstrate that the same measurement can be used to sense a time-varying magnetic field continuously with quantum enhancement, where deep learning model is employed for the inference process. Finally, I will discuss prospects of continuous measurements of non-commuting variables, and hybrid squeezing of different types of spins.
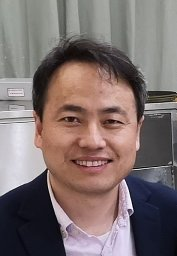
Exploring the fundamental structure and basic laws of the universe constitutes an essential drive to physicists. The studies of ultracold atoms have built a bridge between the principles of microscopic world and condensed matter physics. One can build and manipulate synthetic quantum material to simulate strongly correlated quantum many-body system with microscopic techniques to solve formidable tasks for the state-of-the-art supercomputers. I will introduce our recent research on one of such synthetic quantum material, the lattice gauge theory (LGT). We implemented a U(1) LGT Hamiltonian with ultracold atoms trapped in optical lattices and studied the relevant properties of gauge invariance, thermalization dynamics and quantum criticality [1-6].
References
- Han-Ning Dai et al. Four-body ring-exchange interactions and anyonic statistics within a minimal toric-code Hamiltonian. Nature Physics 13, 1195 (2017).
- Bing Yang et al. Observation of gauge invariance in a 71-site Bose-Hubbard quantum simulator. Nature 587, 392 (2020).
- Zhao-Yu Zhou et al. Thermalization dynamics of a gauge theory on a quantum simulator. Science 377, 311 (2022).
- Guo-Xian Su et al. Observation of many-body scarring in a Bose-Hubbard quantum simulator. Phys. Rev. Res. 5, 023010 (2023).
- Han-Yi Wang et al. Interrelated thermalization and quantum criticality in a lattice gauge simulator, Phys. Rev. Lett. 131, 050401 (2023).
- Wei-Yong Zhang et al. Observation of microscopic confinement dynamics by a tunable topological angle, arXiv:2306.11794.
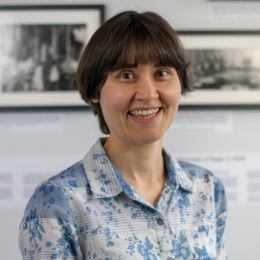
Precise atomic spectroscopy has played a pivotal role in advancing our knowledge of physics. With the emergence of laser cooling and trapping techniques alongside stable light sources, an exceedingly high level of spectroscopic precision has been attained. More recent developments have extended this ultrahigh precision, including atomic clock technologies, to more intricate quantum systems such as diatomic molecules. This extension enables the characterization of molecular degrees of freedom, such as vibrational motion, with a level of resolution approaching that of atomic clocks. Illuminating previously unknown molecular properties, this capability also suggests interesting avenues for exploring fundamental aspects of physical interactions, including the refinement of laboratory based tests probing Newtonian gravity at the nanometer scale.
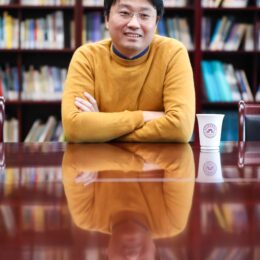
Quantum critical states are manifestations of strong correlations in quantum many-body systems, usually occurring in low dimensions or nearby quantum critical points. Quantum critical states also widely exist in cold atom systems. Examples include one-dimensional cold atomic gases, quantum critical points in optical lattice and Rydberg atom arrays. In this talk, I will discuss several unique cold atom approaches to reveal novel properties of quantum critical states, such as their response to controlled dissipations and reconfigurable geometries and their connection to quantum many-body scars.
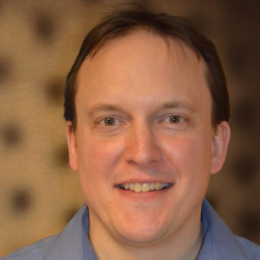
The Hubbard model of attractively interacting fermions provides a paradigmatic setting for fermion pairing, featuring a crossover between Bose-Einstein condensation (BEC) of tightly bound pairs and Bardeen-Cooper-Schrieffer (BCS) superfluidity of long-range Cooper pairs, and a “pseudo-gap” region where pairs form already above the superfluid critical temperature. We directly observe the non-local nature of fermion pairing in a Hubbard lattice gas, employing spin- and density-resolved imaging of ∼1000 fermionic 40K atoms under a bilayer microscope. In the strongly correlated regime, the fermion pair size is found to be on the order of the average interparticle spacing.
When spins are imbalanced, not every fermion can find a partner, resulting in competition between pairing, charge order and magnetism. One candidate ground state in this regime is the Fulde-Ferrell-Larkin-Ovchinnikov (FFLO) state, a spatially modulated superfluid hosting a crystal of excess spins. As a first hint at new order awaiting at lower temperatures, we find that excess spins become attracted by neighboring pairs beyond a critical spin imbalance.
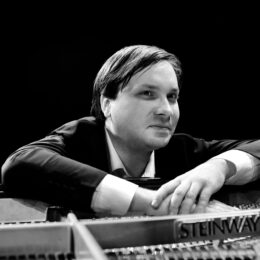
British-Ukrainian pianist Alexei Grynyuk performs throughout the world appearing in the most prestigious concert halls and music festivals. He has performed recitals at the Verbier Music Festival in Switzerland, Newport Music Festival in USA, “Musical Kremlin” Festival in Moscow, Duszniki Chopin Festival in Poland, International Keyboard Festival in New York among many others. The venues include Wigmore Hall and the South Bank Centre in London, Salle Cortot and Salle Gaveau in Paris, Great Hall of Moscow Conservatoire, Concertgebouw in Amsterdam.
Programme:
- Beethoven 32 variations in C minor (WoO 80)
- Schubert sonata No 21 in B flat (D960)
Note – you must have a ticket to attend this event – you can book a ticket here. Only use this option if you did not book a ticket while registering for the conference.
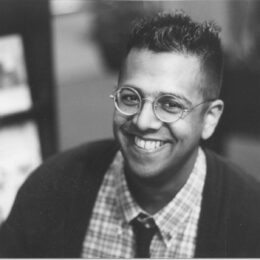
Join Simon Singh, on a whistle-stop tour through two decades of his bestselling books. Fermat’s Last Theorem looks at one of the biggest mathematical puzzles of the millennium; The Code Book shares the secrets of cryptology; Big Bang explores the history of cosmology; Trick or Treatment asks some hard questions about alternative medicine; The Simpsons and Their Mathematical Secrets explains how TV writers, throughout the cartoon’s 25-year history, have smuggled in mathematical jokes. Simon will also talk about his current efforts to increase the number and diversity of excellent mathematicians and physicists.
Note – you must have a ticket to attend this event – you can book a ticket here.